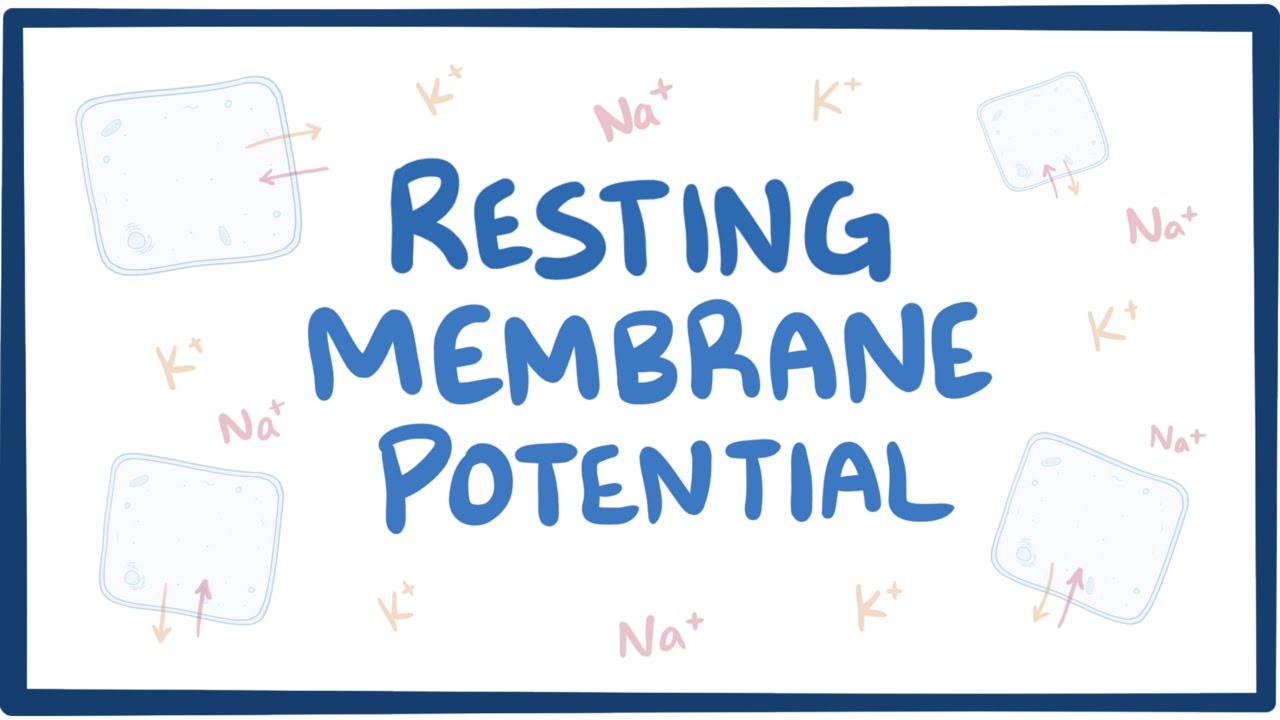
Create articles from any YouTube video or use our API to get YouTube transcriptions
Start for freeThe Basics of Cell Membrane Potential
Every cell in the human body is encased in a membrane that acts as a barrier between the internal and external environments. This membrane plays a crucial role in maintaining the cell's electrical balance, which is fundamental to many cellular processes. At the heart of this balance is the cell membrane potential, a concept that's essential to understand for anyone interested in cellular biology or neuroscience.
The Cell Membrane: A Selective Barrier
The cell membrane is not just a simple wall; it's a complex structure that selectively allows certain substances to pass through while blocking others. This selective permeability is key to maintaining the different concentrations of ions inside and outside the cell. The main players in this ionic balance are:
- Sodium (Na+)
- Potassium (K+)
- Chloride (Cl-)
- Calcium (Ca2+)
Generally, there's a higher concentration of sodium, chloride, and calcium outside the cell, while potassium and various negatively charged molecules (anions) are more concentrated inside the cell.
The Sodium-Potassium Pump: The Cell's Workhorse
One of the most important mechanisms for maintaining these concentration differences is the sodium-potassium pump. This protein complex uses energy in the form of ATP to actively transport ions across the cell membrane. For every cycle:
- 3 sodium ions are pumped out of the cell
- 2 potassium ions are pumped into the cell
This process is crucial for establishing and maintaining the concentration gradients that drive many cellular processes.
Understanding Ion Gradients
Let's take a closer look at potassium as an example to understand how ion gradients work:
- Inside the cell: ~150 mMol/L of potassium
- Outside the cell: ~5 mMol/L of potassium
This significant difference in concentration creates a strong gradient that naturally pushes potassium ions to move out of the cell. However, ions can't simply pass through the lipid bilayer of the cell membrane. Instead, they rely on specific protein channels to cross the membrane.
Potassium Channels: Leak Channels and Inward Rectifiers
Potassium ions can move across the membrane through two types of channels:
- Potassium leak channels
- Inward rectifier channels
These channels allow potassium to flow down its concentration gradient, out of the cell. As potassium ions (which are positively charged) leave the cell, they leave behind unpaired negative charges. This process gradually builds up a negative charge inside the cell.
The Electrostatic Gradient
As the negative charge inside the cell increases, it starts to attract positively charged potassium ions back into the cell. This attraction is called the electrostatic gradient. It's important to note that this gradient is established with the movement of relatively few ions, so it doesn't significantly affect the overall concentration gradient.
Equilibrium Potential: Balancing Act
The point at which the concentration gradient pushing potassium out of the cell exactly balances the electrostatic gradient pulling potassium back into the cell is called the equilibrium potential or Nernst potential. For potassium, this equilibrium potential is approximately -92 mV.
In other words, a negative charge of 92 mV inside the cell is required to balance the concentration gradient pushing potassium out of the cell.
The Nernst Equation: Calculating Equilibrium Potentials
The Nernst equation is a formula used to calculate the equilibrium potential for any ion. It takes into account the concentration gradient of the ion and the temperature. The general form of the Nernst equation is:
For ions with a single charge (like sodium or potassium): Vm = 61.5 * log([ion]outside / [ion]inside)
For ions with a double charge (like calcium): Vm = 30.75 * log([ion]outside / [ion]inside)
Where:
- Vm is the membrane potential
- [ion] represents the concentration of the ion
Equilibrium Potentials for Different Ions
Using the Nernst equation and typical ion concentrations found in cells, we can calculate the equilibrium potentials for the main ions affecting the cell's membrane potential:
- Potassium (K+): -92 mV
- Sodium (Na+): +67 mV
- Chloride (Cl-): -86 mV (note the sign flip due to negative charge)
- Calcium (Ca2+): +123 mV
These values represent the membrane potential required to balance the concentration gradient for each ion.
Resting Membrane Potential: A Weighted Average
The actual resting membrane potential of a cell is not equal to any single ion's equilibrium potential. Instead, it's a weighted average of all the equilibrium potentials, based on how permeable the membrane is to each ion.
In most cells, the membrane is most permeable to potassium, so the resting membrane potential tends to be closest to potassium's equilibrium potential. However, the other ions do contribute to the overall potential.
Calculating Resting Membrane Potential
To calculate the resting membrane potential, we need to know:
- The equilibrium potential for each ion
- The relative permeability of the membrane to each ion
For example, if we assume the following permeabilities:
- Potassium: 90%
- Calcium: 1%
- Sodium: 1%
- Chloride: 8%
We can calculate the resting membrane potential as follows:
(0.90 * -92 mV) + (0.01 * 123 mV) + (0.01 * 67 mV) + (0.08 * -86 mV) = -86 mV
This calculation gives us a resting membrane potential of -86 mV, which is indeed close to potassium's equilibrium potential due to the high permeability of the membrane to potassium.
Factors Affecting Resting Membrane Potential
Several factors can influence a cell's resting membrane potential:
-
Ion concentrations: Changes in the concentration of ions inside or outside the cell can shift the equilibrium potentials.
-
Membrane permeability: The number and type of ion channels in the membrane can affect how easily different ions can cross the membrane.
-
Temperature: The Nernst equation includes a temperature component, so changes in temperature can affect equilibrium potentials.
-
pH: Changes in pH can affect the function of ion channels and pumps, indirectly affecting membrane potential.
-
Cellular metabolism: The sodium-potassium pump requires ATP, so changes in cellular energy production can affect ion gradients.
Importance of Membrane Potential in Cellular Functions
The resting membrane potential is not just an interesting physical property of cells; it plays crucial roles in many cellular functions:
1. Nerve Signal Transmission
In neurons, changes in membrane potential are the basis for generating and transmitting electrical signals. Action potentials, the rapid changes in membrane potential that propagate along nerve fibers, are the fundamental mechanism of neural communication.
2. Muscle Contraction
Changes in membrane potential trigger the release of calcium ions in muscle cells, initiating the process of muscle contraction.
3. Secretion in Gland Cells
Many secretory cells use changes in membrane potential to trigger the release of hormones or other substances.
4. Nutrient Transport
Some transport processes across the cell membrane are driven by the electrical gradient established by the membrane potential.
5. Cell Volume Regulation
The distribution of ions across the membrane, which contributes to the membrane potential, also plays a role in regulating cell volume.
Measuring Membrane Potential
Scientists can measure the membrane potential of cells using various techniques:
-
Microelectrodes: Tiny glass pipettes filled with a conductive solution can be inserted into cells to directly measure the potential difference across the membrane.
-
Patch clamp technique: This advanced method allows researchers to study individual ion channels by isolating a small patch of cell membrane.
-
Voltage-sensitive dyes: These fluorescent molecules change their properties based on the membrane potential, allowing for non-invasive measurements.
Membrane Potential in Different Cell Types
While we've discussed general principles, it's important to note that different types of cells can have different resting membrane potentials and ion permeabilities:
-
Neurons: Typically have a resting potential around -70 mV and are specialized for rapid changes in membrane potential.
-
Muscle cells: Have a resting potential similar to neurons but are optimized for coupling electrical signals to mechanical contraction.
-
Epithelial cells: Often have more positive resting potentials, around -50 to -70 mV, and use their membrane potential for transport processes.
-
Plant cells: Can have much more negative resting potentials, sometimes as low as -200 mV, due to the activity of proton pumps in their cell membranes.
Disorders Related to Membrane Potential
Disruptions in the mechanisms that maintain proper membrane potential can lead to various disorders:
-
Channelopathies: Genetic disorders affecting ion channels can lead to conditions like certain forms of epilepsy, migraine, or cardiac arrhythmias.
-
Hypokalemia and Hyperkalemia: Abnormal levels of potassium in the blood can significantly affect membrane potentials throughout the body, potentially leading to muscle weakness or heart rhythm disturbances.
-
Cystic Fibrosis: This genetic disorder affects chloride channels, leading to disrupted ion transport across cell membranes.
-
Certain poisonings: Some toxins, like tetrodotoxin found in pufferfish, work by blocking sodium channels and disrupting normal membrane potential dynamics.
Future Directions in Membrane Potential Research
Research into cell membrane potentials continues to be an active area of study:
-
Optogenetics: This emerging field combines genetics and optics to control membrane potential in specific cells using light, offering new ways to study and potentially treat neurological disorders.
-
Bioelectricity in development and regeneration: There's growing interest in how bioelectric signals, including membrane potentials, guide embryonic development and tissue regeneration.
-
Artificial cells: Engineers are working on creating synthetic cells with controllable membrane potentials, which could have applications in drug delivery or biosensing.
-
Computational modeling: Advanced computer models are being developed to simulate the complex interplay of ions and channels that determine membrane potential.
Conclusion
The cell membrane potential is a fundamental aspect of cellular physiology, arising from the interplay of ion concentrations, membrane permeability, and active transport processes. Understanding these principles is crucial for grasping how cells maintain their internal environment, communicate with each other, and respond to external stimuli.
From the basics of ion gradients to the complexities of the Nernst equation and the calculation of resting membrane potentials, this field offers rich insights into the functioning of living systems. As research continues, our understanding of membrane potentials will undoubtedly lead to new insights in biology and medicine, potentially opening up novel therapeutic approaches for a wide range of conditions.
Whether you're a student of biology, a medical professional, or simply curious about the intricacies of life at the cellular level, the study of membrane potentials offers a fascinating window into the fundamental processes that keep our cells - and us - alive and functioning.
Article created from: https://youtu.be/hk09AkV5_Kc?si=uUf3BZn5aFA_mp0O