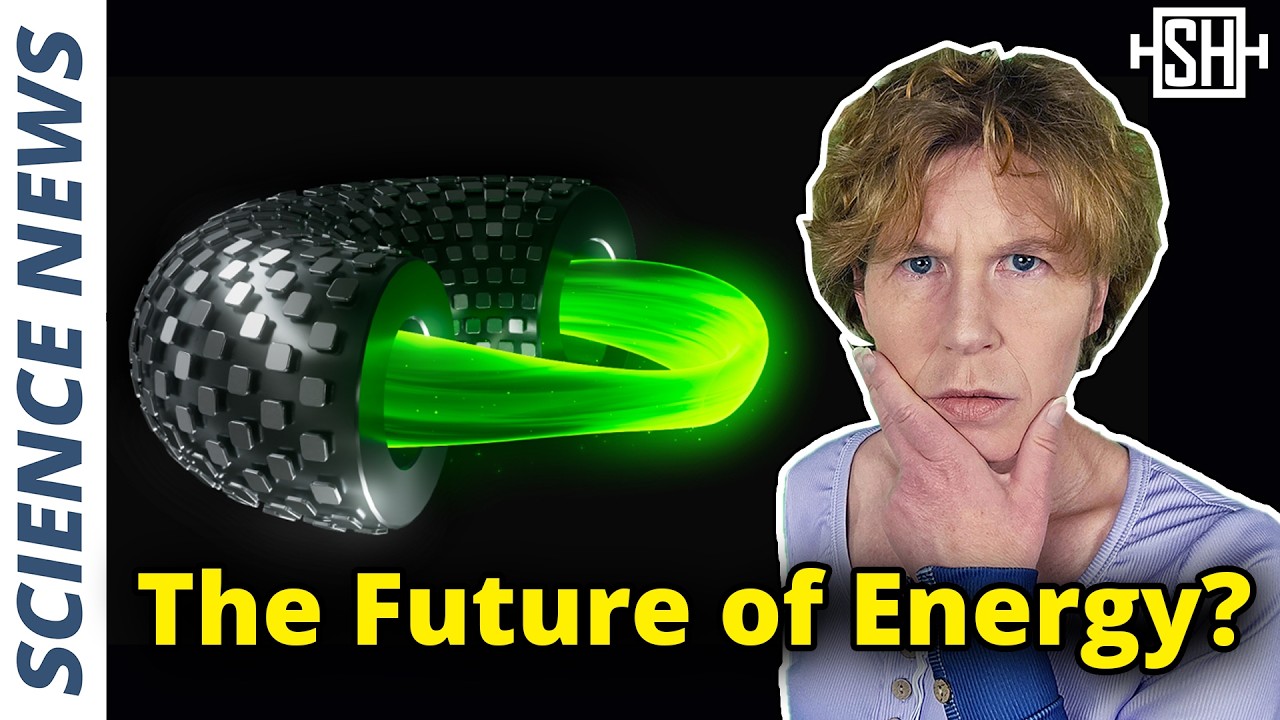
Create articles from any YouTube video or use our API to get YouTube transcriptions
Start for freeThe Promise of Nuclear Fusion
Nuclear fusion has long been hailed as the ultimate solution to our energy needs. It offers the potential for near-limitless, clean, and safe power generation. Unlike traditional nuclear fission, fusion produces minimal radioactive waste and poses no risk of meltdown. The challenge, however, lies in controlling the incredibly hot plasma required for fusion reactions.
The Tokamak Approach
For decades, the most researched approach to fusion has been the tokamak design. Tokamaks use a combination of magnetic and electric fields to confine plasma within a toroidal (donut-shaped) chamber. While tokamaks have made significant progress, they face several challenges:
- Plasma instability
- Pulsed operation
- Difficulty in scaling up
Recent achievements in tokamak technology include:
- China's EAST tokamak: Controlled plasma for over 1,000 seconds
- France's WEST tokamak: Maintained plasma for over 1,300 seconds
- UK's JET (Joint European Torus): Produced a record-breaking 69 megajoules of energy
Despite these impressive milestones, tokamaks have yet to achieve net energy gain from fusion reactions. They also suffer from frequent plasma disruptions, which can damage the reactor and hinder progress.
The Rise of Stellarators
In recent years, an alternative fusion reactor design has gained significant attention: the stellarator. Stellarators offer several advantages over tokamaks:
- Improved plasma stability
- Continuous operation
- Potential for easier scaling
The key difference between stellarators and tokamaks lies in how they generate the magnetic fields necessary to confine the plasma. While tokamaks rely on a combination of external magnets and a current running through the plasma itself, stellarators use only external magnets. These magnets are twisted into complex three-dimensional shapes, creating a more stable magnetic field configuration.
Historical Challenges
Despite their potential advantages, stellarators have historically been less pursued than tokamaks due to two main challenges:
- Design complexity: Calculating the precise magnetic field configurations required supercomputers and advanced simulations.
- Manufacturing difficulty: The intricate shapes of the magnetic coils often necessitated hand-crafting, making large-scale production impractical.
Recent Breakthroughs
Advances in technology have largely overcome these obstacles:
- Machine learning algorithms now assist in optimizing magnetic field designs.
- High-precision engineering and advanced manufacturing techniques enable the production of complex coil shapes.
These developments have led to a resurgence of interest in stellarators, with several companies making significant strides in the technology.
Thea Energy's Planar Coil Stellarator
In January, US-based Thea Energy published four peer-reviewed papers outlining their innovative approach to stellarator design. Their concept, called the planar coil stellarator, aims to simplify the complex 3D coils typically associated with stellarators.
Key Features
- Simpler coil design: Uses planar (flat) coils instead of intricate 3D shapes.
- Software-driven field shaping: Employs computer algorithms to steer the magnetic field, shifting the complexity from hardware to software.
- Prototype success: In March, Thea Energy tested a prototype of their planar coils, achieving magnetic fields over 3 Tesla – sufficient for confining fusion-relevant plasma.
This approach could significantly reduce manufacturing costs and complexity, potentially accelerating the development of commercial fusion reactors.
Proxima Fusion's Stellaris
German startup Proxima Fusion made waves in March with the publication of their design plans for a commercial stellarator power plant named Stellaris.
Notable Aspects
- Open-source approach: Proxima Fusion released the code used to calculate plasma behavior, fostering collaboration and transparency in the fusion community.
- Ambitious timeline: The company aims to build a demonstration device by 2031.
- Focus on magnetic coils: Proxima Fusion is actively working on optimizing the magnetic coil designs crucial for stellarator performance.
By open-sourcing their code, Proxima Fusion is contributing to the collective knowledge in fusion research, potentially accelerating progress across the entire field.
Type One Energy's Infinity 2
Just a week after Proxima Fusion's announcement, Type One Energy released several papers detailing their plans for a pilot fusion plant called Infinity 2.
Key Points
- Comprehensive design: The papers present a detailed, self-consistent model for building a stellarator capable of producing 800 megawatts of fusion power.
- Commercial focus: Type One Energy's design is geared towards a practical, operational power plant.
- Ambitious goal: The company aims to have a power plant operational by the mid-2030s.
Type One Energy's detailed plans demonstrate the growing maturity of stellarator technology and its potential for commercial application.
The Significance of Recent Announcements
The near-simultaneous release of design plans by three different stellarator companies is noteworthy for several reasons:
- Rapid progress: It highlights the accelerating pace of development in stellarator technology.
- Growing confidence: Companies are willing to share detailed plans, indicating a high level of confidence in their approaches.
- Collaborative spirit: The openness of these companies suggests a desire to advance the field as a whole, not just their individual projects.
- Investor appeal: Publishing detailed plans helps build credibility and attract potential investors.
Advantages of Stellarators Over Tokamaks
As stellarator technology matures, several advantages over tokamaks become apparent:
- Stability: Stellarators offer inherently more stable plasma confinement, reducing the risk of disruptions that plague tokamaks.
- Continuous operation: Unlike tokamaks, which operate in pulses, stellarators can run continuously, simplifying power generation.
- Scalability: The absence of a plasma current makes stellarators potentially easier to scale up to commercial size.
- Efficiency: Advanced stellarator designs promise improved energy efficiency compared to tokamaks.
- Maintenance: The more stable plasma in stellarators may lead to reduced wear on reactor components, lowering maintenance costs.
Challenges Facing Stellarator Development
Despite recent progress, stellarators still face several challenges:
- Complexity: While simplified designs like Thea Energy's planar coil approach help, stellarators remain complex machines.
- Cost: The precision engineering required for stellarators can be expensive, though economies of scale may reduce costs over time.
- Heat exhaust: Managing the intense heat produced by fusion reactions remains a significant engineering challenge.
- Neutron damage: As with all fusion designs, protecting reactor components from neutron bombardment is crucial for long-term operation.
- Fuel cycle: Developing efficient methods for breeding and handling tritium fuel is essential for commercial viability.
The Role of Advanced Materials in Fusion
Progress in materials science plays a crucial role in advancing fusion technology, including stellarators:
- High-temperature superconductors: These materials could enable more powerful and efficient magnetic fields.
- Neutron-resistant alloys: Developing materials that can withstand intense neutron bombardment is crucial for reactor longevity.
- Advanced ceramics: These materials may offer superior heat resistance and electrical insulation properties.
- Nanostructured materials: Engineered at the nanoscale, these materials could provide unique properties beneficial for fusion reactors.
Computer Simulation and AI in Stellarator Design
The resurgence of stellarators owes much to advancements in computational power and artificial intelligence:
- Plasma physics modeling: Sophisticated simulations allow researchers to predict and optimize plasma behavior.
- Magnetic field optimization: AI algorithms can rapidly iterate through countless magnetic field configurations to find optimal designs.
- Engineering simulations: Advanced modeling helps in designing reactor components to withstand the extreme conditions of fusion.
- Operational control: AI may play a crucial role in the real-time control of fusion reactors, maintaining optimal conditions for sustained reactions.
The Potential Impact of Successful Fusion
If stellarators or other fusion technologies achieve commercial viability, the impact could be transformative:
- Energy abundance: Fusion could provide virtually limitless clean energy, addressing global energy needs.
- Climate change mitigation: As a carbon-free energy source, fusion could play a crucial role in combating climate change.
- Economic growth: The fusion industry could create numerous high-skilled jobs and drive technological innovation.
- Geopolitical stability: Reducing dependence on fossil fuels could alter global power dynamics.
- Space exploration: Compact fusion reactors could revolutionize space travel, enabling long-distance missions.
The Road to Commercial Fusion
While recent progress is encouraging, several steps remain before fusion becomes a commercial reality:
- Achieving net energy gain: Demonstrating that more energy can be produced than is consumed to initiate and sustain fusion reactions.
- Scaling up: Moving from experimental reactors to commercial-scale power plants.
- Cost reduction: Bringing down the cost of fusion power to compete with other energy sources.
- Regulatory framework: Developing appropriate safety and regulatory standards for fusion power plants.
- Infrastructure development: Building the necessary supply chains and training a skilled workforce for the fusion industry.
Conclusion
The rapid progress in stellarator technology, exemplified by recent announcements from Thea Energy, Proxima Fusion, and Type One Energy, marks a significant milestone in the quest for practical nuclear fusion. These developments suggest that stellarators may indeed be the key to unlocking the potential of fusion energy.
The willingness of private companies to publish their designs and, in some cases, their code, demonstrates a collaborative approach that could accelerate progress across the entire field. This openness, combined with advancements in computer simulation, AI, and materials science, is driving fusion research forward at an unprecedented pace.
While challenges remain, the potential benefits of fusion energy – clean, safe, and virtually limitless power – make it a goal worth pursuing. As stellarator technology continues to mature, we may be witnessing the dawn of a new era in energy production, one that could revolutionize our approach to power generation and help address some of the most pressing challenges facing our planet.
The coming years will be crucial in determining whether stellarators can deliver on their promise. If successful, they could provide a solution to our energy needs that is truly out of this world – or rather, inspired by the stars themselves.
Article created from: https://www.youtube.com/watch?v=e7hw0aC1BbI