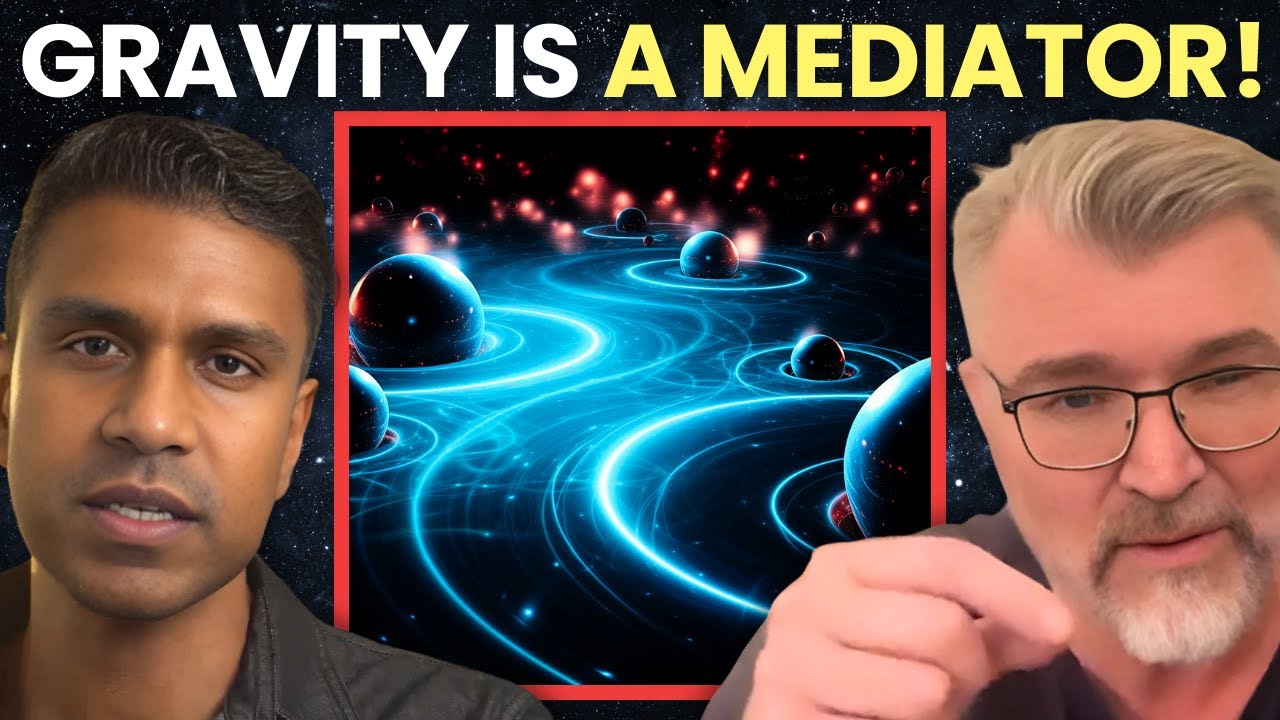
Create articles from any YouTube video or use our API to get YouTube transcriptions
Start for freeThe Nature of Quantum Gravity
The concept of quantum gravity has long been a subject of fascination and intense study in the world of theoretical physics. At its core, quantum gravity seeks to reconcile two fundamental pillars of modern physics: quantum mechanics and general relativity. While these theories have been incredibly successful in their respective domains, they seem to be incompatible when it comes to describing gravity at the quantum scale.
One of the key questions in this field is whether gravity itself can exist in a state of superposition, much like other quantum entities. This idea challenges our classical understanding of spacetime and opens up new avenues for exploring the fundamental nature of reality.
Understanding Superposition in Quantum Gravity
Superposition is a fundamental principle of quantum mechanics, which states that a quantum system can exist in multiple states simultaneously until it is observed or measured. When we apply this concept to gravity and spacetime itself, we enter a realm of physics that is both fascinating and perplexing.
The idea of spacetime superposition distinguishes a fully quantum theory of gravity from a semi-classical approach that combines classical gravity with quantum mechanics. In a quantum theory of gravity, the geometry of spacetime itself could potentially exist in a superposition of different configurations.
However, it's crucial to note that demonstrating the quantum nature of gravity does not necessarily mean we can easily prepare gravity in a superposition state. There are different aspects of "quantumness" that we need to consider:
- The ability to create entanglement
- The ability to be set in a superposition of classical configurations
Current experimental proposals, such as the Gravitationally Induced Entanglement (GIE) experiment, focus on testing the former aspect - the ability of gravity to mediate entanglement between quantum objects.
The Gravitationally Induced Entanglement (GIE) Experiment
The GIE experiment is a groundbreaking proposal aimed at testing the quantum nature of gravity. Unlike previous suggestions, this experiment doesn't attempt to directly observe gravitons or create superpositions of spacetime. Instead, it focuses on gravity's ability to mediate entanglement between two quantum systems.
Here's how the experiment works in principle:
- Two massive quantum objects are placed in close proximity but not touching.
- Each object is put into a superposition of two different spatial locations.
- If gravity is indeed quantum, it should be able to "see" both positions of each object simultaneously.
- This quantum interaction through gravity should lead to entanglement between the two objects.
- By measuring the entanglement between the objects, we can infer whether gravity behaves quantum mechanically.
This approach is ingenious because it bypasses the need to directly detect individual gravitons, which is currently beyond our technological capabilities.
The Significance of Mediation in Quantum Gravity
The concept of mediation is crucial in understanding how gravity might operate at the quantum level. In physics, mediation refers to how forces or interactions are transmitted between objects. For gravity, the question is whether it acts directly between objects (action at a distance) or through a mediating field.
Classical physics, following Newton's theory of gravity, struggled with the idea of instantaneous action at a distance. This concept seemed to violate principles of causality and conservation of energy. The introduction of fields in physics, pioneered by Faraday and formalized by Maxwell and Einstein, resolved many of these issues.
In the context of quantum gravity, mediation becomes even more critical. Quantum objects can exist in superpositions, effectively being in multiple places at once. For gravity to interact with such objects consistently, it must be able to respond to all possible positions simultaneously. This requirement strongly suggests that gravity itself must have quantum properties.
The GIE experiment tests this mediating property of gravity. If gravity can indeed mediate entanglement between two quantum objects, it would provide strong evidence for its quantum nature.
Challenges in Testing Quantum Gravity
Testing quantum gravity poses significant challenges due to the extremely weak nature of gravitational interactions at the scales we can currently probe. Some of these challenges include:
-
Graviton Detection: Unlike photons, which are relatively easy to detect individually, gravitons (the hypothetical particles that mediate the gravitational force) are incredibly elusive. Freeman Dyson's work suggests that detecting individual gravitons might be practically impossible.
-
Energy Scales: The energy scales at which quantum gravity effects become significant are far beyond what we can achieve in particle accelerators or observe in cosmic rays.
-
Interference Experiments: While we can perform interference experiments with photons to demonstrate their wave-like nature, doing the same with gravity is currently unfeasible.
-
Decoherence: Even if we could create superpositions of spacetime, they might decohere too quickly to be observed due to gravity's universal coupling to all matter and energy.
Despite these challenges, the GIE experiment offers a promising approach to probe the quantum nature of gravity without needing to directly manipulate or observe gravitons.
Implications of Quantum Gravity
If gravity is indeed quantum in nature, as the GIE experiment aims to test, it would have profound implications for our understanding of the universe:
-
Unification of Forces: It would bring us closer to a unified theory of all fundamental forces, a long-standing goal in physics.
-
Early Universe: Quantum gravity is expected to play a crucial role in understanding the very early universe and potentially what happened before the Big Bang.
-
Black Holes: It could help resolve paradoxes related to black hole information and evaporation.
-
Spacetime Structure: At the smallest scales, spacetime might have a discrete, granular structure rather than being continuous.
-
Quantum Cosmology: It could lead to new models of the universe that incorporate quantum effects on a cosmic scale.
Beyond GIE: Future Directions in Quantum Gravity Research
While the GIE experiment represents a significant step forward, it's important to recognize that it tests only one aspect of quantum gravity - its ability to mediate entanglement. Future research in quantum gravity might explore:
-
Spacetime Superposition: Developing experiments or observations that could directly test for superpositions of spacetime geometries.
-
Quantum Gravity in Cosmology: Looking for signatures of quantum gravitational effects in the cosmic microwave background or other cosmological observations.
-
Analogue Gravity Systems: Using analogues in condensed matter systems to study aspects of quantum gravity that are otherwise inaccessible.
-
Quantum Gravity and Information: Exploring the connections between quantum gravity, information theory, and the holographic principle.
-
Experimental Improvements: Enhancing the sensitivity and scale of experiments like GIE to probe gravity at increasingly smaller scales.
The Role of Theoretical Frameworks
While experiments like GIE are crucial, theoretical work in quantum gravity continues to play a vital role. Various approaches to quantum gravity have been developed, including:
-
String Theory: Proposes that all particles and forces arise from the vibrations of tiny, one-dimensional strings.
-
Loop Quantum Gravity: Attempts to quantize spacetime itself, suggesting a granular structure at the smallest scales.
-
Causal Dynamical Triangulations: A computational approach to quantum gravity that builds spacetime from simple geometric building blocks.
-
Asymptotic Safety: Suggests that gravity might be renormalizable when treated as an effective field theory.
-
Emergent Gravity: Proposes that gravity might not be a fundamental force but emerges from more basic quantum processes.
These theoretical frameworks provide different perspectives on how to reconcile quantum mechanics with gravity and offer predictions that future experiments might test.
The Importance of Conservation Laws
One of the key motivations for quantum gravity comes from the need to maintain fundamental conservation laws. In classical physics, fields play a crucial role in ensuring the conservation of energy, momentum, and angular momentum in interactions between distant objects.
For example, when a planet moves in its orbit around the sun, its kinetic energy changes. In a classical field theory, this energy is stored in the gravitational field and propagates at the speed of light, ensuring that energy is conserved at all times.
In quantum mechanics, where objects can exist in superpositions of different positions, the gravitational field must be able to respond to all possible positions simultaneously to maintain these conservation laws. This requirement strongly suggests that gravity itself must have quantum properties.
The ability of a quantum theory of gravity to uphold these conservation laws exactly, rather than just statistically or on average, is seen as a significant advantage over semi-classical approaches.
Locality and Mediation in Physics
The concept of locality is fundamental to our understanding of physics. It states that interactions occur at specific points in space and time, and effects propagate from these points at finite speeds, never exceeding the speed of light.
This principle is closely tied to the idea of mediation. In modern physics, forces are understood to be mediated by fields or particles:
- Electromagnetism is mediated by photons
- The strong nuclear force is mediated by gluons
- The weak nuclear force is mediated by W and Z bosons
Gravity, in Einstein's general relativity, is mediated by the curvature of spacetime itself. In a quantum theory of gravity, this mediation would likely be described in terms of gravitons, the hypothetical particles of the gravitational field.
The GIE experiment assumes this mediated nature of gravity, which is consistent with our understanding of other fundamental forces. This assumption is crucial because it allows us to test the quantum properties of gravity without needing to directly observe gravitons.
Limitations of the GIE Experiment
While the GIE experiment is a powerful probe of the quantum nature of gravity, it's important to understand its limitations:
-
No Direct Superposition: The experiment doesn't directly create or observe superpositions of spacetime or gravitational fields.
-
Classical Mediation: It can't rule out theories where gravity is classical but non-locally mediated.
-
Energy Scale: The experiment operates at relatively low energies compared to where full quantum gravity effects are expected to dominate.
-
Interpretation: The results will require careful interpretation to distinguish quantum gravitational effects from other possible explanations.
-
Technical Challenges: Achieving the necessary level of isolation and sensitivity for the experiment is extremely challenging.
Despite these limitations, the GIE experiment represents a significant step forward in our ability to probe the quantum nature of gravity.
Conclusion: The Road Ahead for Quantum Gravity
The quest to understand quantum gravity remains one of the most exciting and challenging frontiers in theoretical physics. The proposed GIE experiment offers a promising approach to test some aspects of quantum gravity, potentially providing crucial empirical evidence to guide further theoretical development.
However, fully understanding quantum gravity will likely require a multifaceted approach, combining:
- Innovative experiments like GIE
- Astronomical observations of extreme gravitational environments
- Advanced theoretical frameworks
- Computational simulations and analogue systems
As we continue to push the boundaries of our understanding, we may need to revise our fundamental concepts of space, time, and the nature of reality itself. The journey to unravel the mysteries of quantum gravity promises to be one of the most intellectually rewarding endeavors in the history of science, potentially revolutionizing our understanding of the universe at its most fundamental level.
Article created from: https://www.youtube.com/watch?v=S22p50SFf3g