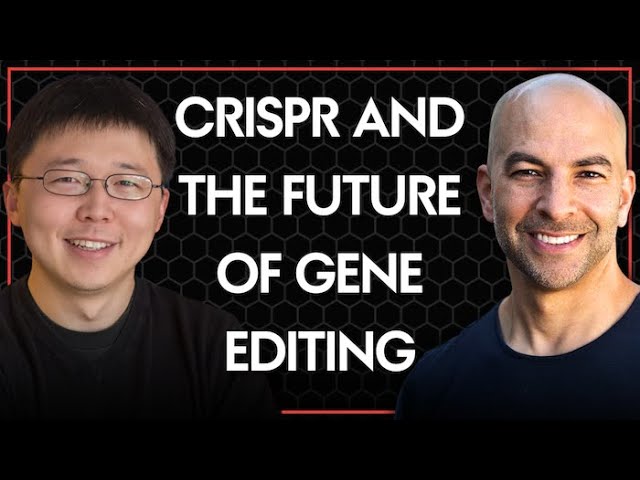
Create articles from any YouTube video or use our API to get YouTube transcriptions
Start for freeThe Origins of CRISPR
The story of CRISPR gene editing technology begins in the 1980s, when Japanese researchers discovered unusual repeating DNA sequences in bacteria. These sequences, which came to be known as Clustered Regularly Interspaced Short Palindromic Repeats (CRISPR), were initially a mystery.
It wasn't until the early 2000s that scientists began to unravel their purpose. Spanish researcher Francisco Mojica made a crucial breakthrough when he realized that the non-repeating spacer sequences between the repeats matched viral DNA. This suggested CRISPR was part of a bacterial immune system against viruses.
Further research revealed that bacteria use CRISPR sequences along with CRISPR-associated (Cas) proteins to detect and destroy viral DNA. When a virus infects a bacterium, the CRISPR system incorporates a piece of the viral DNA into its own genome. This allows the bacterium to recognize and quickly destroy that virus if it attacks again in the future.
From Bacterial Immunity to Gene Editing Tool
The potential for CRISPR to be used as a gene editing tool in other organisms was recognized in the early 2010s. Researchers realized that by providing an engineered guide RNA matching a target DNA sequence, along with the Cas9 enzyme, they could direct cuts to specific locations in a genome.
This opened up unprecedented possibilities for precise genetic modification. Unlike earlier gene editing techniques that were cumbersome and inefficient, CRISPR-Cas9 offered a relatively simple, fast and inexpensive method.
Dr. Feng Zhang, a pioneer in the development of CRISPR technology, explains:
"CRISPR is like the smartphone - you can load software onto it to recognize different genes. The software is the CRISPR RNA. These RNAs are very easy to chemically synthesize and you can define the gene by reading off the sequence of the gene, which is already completed through the Human Genome Project."
Applications in Research and Medicine
CRISPR has rapidly become an indispensable tool in biological research, allowing scientists to easily modify genes in cells and model organisms to study their function. But the most exciting potential lies in using CRISPR to treat genetic diseases in humans.
Some of the diseases that may be amenable to CRISPR-based therapies include:
- Blood disorders like sickle cell anemia
- Eye diseases causing blindness
- Neurodegenerative conditions like Huntington's disease
- Inherited metabolic disorders
Clinical trials are already underway for CRISPR treatments targeting diseases like sickle cell anemia. The approach involves removing a patient's blood stem cells, editing them with CRISPR to correct the genetic defect, and then returning them to the patient.
For sickle cell disease, rather than directly correcting the mutation, researchers are using CRISPR to reactivate fetal hemoglobin production. This can compensate for the defective adult hemoglobin and reduce symptoms.
Liver diseases are another promising target, as lipid nanoparticles can be used to deliver CRISPR components directly to liver cells in the body. Trials are ongoing for using this approach to knock out the PCSK9 gene and lower cholesterol levels.
Eye diseases are also being targeted, as the eye is easily accessible for direct injection of CRISPR therapies. Clinical trials are testing CRISPR-based treatments for a form of inherited blindness called Leber congenital amaurosis.
Challenges and Limitations
Despite the immense potential, there are still significant challenges to overcome before CRISPR can be widely used as a medical treatment:
Delivery
Getting CRISPR components into the right cells in the body remains difficult for many tissues and organs. As Dr. Zhang notes:
"The payload technology has come a long way...but the bottleneck is how do we put these really powerful payloads into the right cells in the right tissue in the body."
Viral vectors and lipid nanoparticles work for some tissues, but better delivery methods are needed to reach others.
Efficiency
The editing efficiency of CRISPR varies depending on the target site and cell type. For some applications, very high editing rates are needed to achieve a therapeutic effect.
Off-target effects
There is always a risk of CRISPR making unintended edits at other sites in the genome. Improving the specificity of CRISPR systems remains an active area of research.
Limited ability to insert large DNA sequences
While CRISPR is very good at making small edits or disrupting genes, inserting large new genes remains challenging. As Dr. Zhang explains:
"The Holy Grail would be to be able to insert a gene into anywhere you want precisely and also very efficiently. And to date that ability is still not quite there yet."
Mosaicism
When editing embryos or early stage organisms, it's difficult to ensure all cells are edited identically. This can result in mosaic organisms with a mix of edited and unedited cells.
Ethical Considerations
The power of CRISPR to make heritable changes to the human genome has raised significant ethical concerns. A major controversy erupted in 2018 when a Chinese scientist, He Jiankui, announced he had used CRISPR to edit human embryos that were then used to establish pregnancies.
The resulting twin girls were born with genetic modifications intended to make them resistant to HIV infection. However, the edits were not completely successful and the long-term effects are unknown. There was widespread condemnation from the scientific community over the premature and irresponsible use of CRISPR for human germline editing.
This incident sparked intense debate over if and when human germline editing should be permitted. While there is general agreement that using CRISPR to prevent serious genetic diseases could be ethical if proven safe, many worry about a slippery slope toward "designer babies" enhanced for non-medical traits.
Dr. Zhang offers his perspective on where lines should be drawn:
"I think the things that are very easy to agree on is that if there is an obvious and important medical benefit, especially for inborn errors of metabolism or other genetic mutations, if the technology is there I think it's something that is definitely doable and I think it's okay to improve the lives of those patients."
However, he notes there is still disagreement even on this point, with some arguing against any germline editing. He also points out that for many potential applications, there may be alternatives like preimplantation genetic testing of IVF embryos that could achieve similar goals without the risks of editing.
For enhancements beyond medical necessity, Dr. Zhang sees both scientific and ethical reasons for caution:
"Biology has a lot of compensation. You might make some mutations that make the person smarter but that could also increase cancer risk, or impair athletic capability, or make a person live shorter. Those complicated trade-offs are things that I think people maybe don't fully appreciate."
He also notes the importance of genetic diversity for the resilience of the human species as a whole.
The Future of CRISPR
Despite the challenges and ethical concerns, research on CRISPR technology continues to advance rapidly. Some key areas of ongoing development include:
New CRISPR systems
Researchers are discovering and engineering new CRISPR-associated proteins beyond the commonly used Cas9. For example, Cas13 targets RNA instead of DNA and has applications in diagnostics.
More precise editing
Techniques like base editing and prime editing allow for even more precise genetic changes without making double-strand breaks in DNA.
Improved delivery methods
New approaches are being developed to deliver CRISPR components to a wider range of tissues in the body.
Epigenetic editing
CRISPR systems are being adapted to make reversible changes to gene regulation rather than permanent DNA edits.
AI-assisted design
Machine learning approaches are being used to predict off-target effects and design more effective guide RNAs.
Dr. Zhang is optimistic about the future of the field:
"I'm so excited about all the rapid advances that are happening. Knowledge about biology, about the human body, physiology is accumulating at very rapid pace. Every week there are interesting exciting discoveries being reported and our technologies for studying biological systems are also accelerating."
He envisions a future where AI, robotics, and CRISPR work together to accelerate scientific discovery:
"With the advent of AI and larger computing platforms, we can analyze and learn and build models around those data that are much more powerful and much more predictive and generative than ever before. And that combined with future advances in robotics where we can have automation of experimentation and maybe building a closed loop system where AI and with human intervention can design and iterate our experiments rapidly, I think it's going to accelerate science and medicine and human health beyond our imagination."
Nurturing the Next Generation of Scientists
To realize this exciting future, it's critical to inspire and support the next generation of scientists and engineers. Dr. Zhang credits his own success to dedicated teachers and mentors who nurtured his curiosity from a young age.
He recalls a pivotal moment in 10th grade when he first got to do hands-on molecular biology experiments:
"The very first experiment I did was where the scientist had me put the gene for a jellyfish protein called Green Fluorescent Protein. This is a protein that makes jellyfish glow. And I put this gene into a human cancer cell just using lipid. We wrapped it up with a bit of fat and then it got absorbed by the cell."
"The following day I went to the lab and the scientist took me into this dark microscope room where there was a beam of blue light coming out of the microscope. We put the petri dish with the cells on it and he told me to look into the eyepiece and I saw a field of green cells that were fluorescing, that had taken up this gene. It looked alien to me and that moment just made me feel so inspired about what we were doing."
Dr. Zhang emphasizes the importance of providing similar opportunities to young people today:
"We need to get kids more excited about science. And I think we need an education system to support kids who are curious and who have special interests in science and technical things and to give them opportunities like the ones that I had to really explore those curiosities and interests."
He notes that investing in science education may not pay off immediately, but is crucial for the future:
"This is not an investment that pays off in a year. You've got to be doing this today and then you'll get your payoff in a decade and two decades. And sometimes those investments are really hard for society to make because it's hard to say I got to pay money now for something and I don't get paid back for 10 or 20 years."
Conclusion
CRISPR gene editing technology represents one of the most significant scientific breakthroughs of the 21st century. Its potential applications in medicine, agriculture, and basic research are vast. However, realizing this potential will require overcoming significant technical challenges as well as carefully navigating complex ethical issues.
As the technology continues to advance, it's critical that scientists, policymakers, and the public engage in ongoing dialogue about how to responsibly harness the power of CRISPR. With the right approach, CRISPR could usher in a new era of precision medicine and scientific discovery, dramatically improving human health and our understanding of biology.
At the same time, we must invest in nurturing the next generation of scientific talent. By inspiring young people and providing them with opportunities to explore their curiosity, we can ensure that the incredible potential of CRISPR and other emerging technologies is fully realized in the decades to come.
Article created from: https://www.youtube.com/watch?v=aOl0IDhzLCo