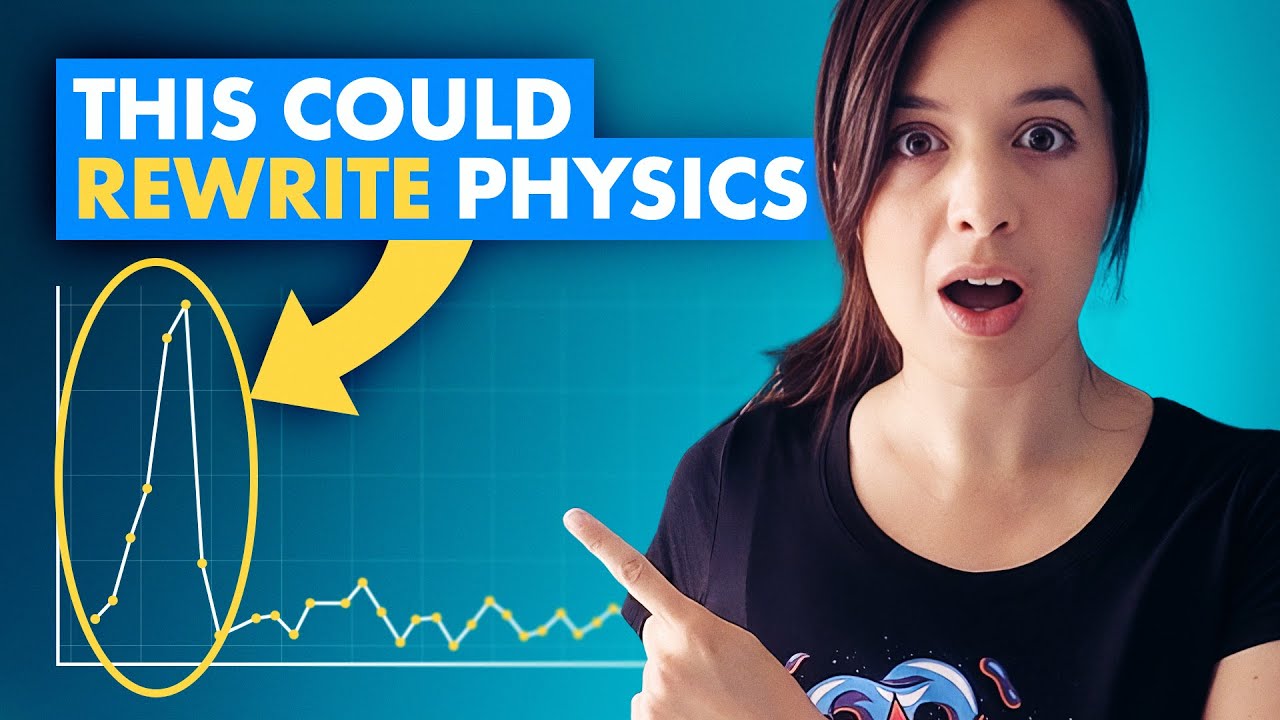
Create articles from any YouTube video or use our API to get YouTube transcriptions
Start for freeThe Groundbreaking Discovery
Scientists have finally detected a signal they've been searching for over two decades - a direct message from the nucleus of the thorium-229 isotope. This breakthrough has profound implications for our understanding of the universe and could help unlock some of the most fundamental laws of physics.
Why This Signal Matters
The detection of this signal is a crucial step towards creating the world's first nuclear clock. This device would be precise enough to detect minute changes in the fundamental constants of the universe - something that has been impossible to measure until now.
Fundamental Constants: The Building Blocks of Our Universe
Fundamental constants are fixed numbers that exist in the universe and set the stage for how the laws of physics work. Some examples include:
- The speed of light
- Boltzmann's constant
- The gravitational constant
Physicists have identified 26 fundamental constants that shape our universe. The current theory of known matter, the Standard Model, treats these numbers as unchanging in both space and time.
The Mystery of Fundamental Constants
One of the most intriguing aspects of fundamental constants is that they can't be predicted from theory. They have to be experimentally measured, and no one knows why they have the values they do. As far as we know, these numbers are simply written into the fabric of the universe.
Questioning the Constancy of Constants
Despite their name, some scientists are beginning to question whether fundamental constants are truly constant. This questioning arises from several observations and theories:
- The "fine-tuning" problem
- Implications of string theory
- Potential interactions with dark matter
The Fine-Tuning Problem
Many of the constants have values that seem perfectly tuned to support life. If some of them were off by even the tiniest amount, life as we know it probably wouldn't exist.
A prime example is the fine structure constant, which has a value of about 1/137. This constant determines the strength of electromagnetism, affecting electricity, magnetism, light, friction, and essentially all of chemistry.
The fine structure constant's value lies in a perfect "sweet spot":
- Strong enough to bind electrons to atoms' nuclei
- Weak enough to allow electrons to interact with other atoms, enabling chemical reactions
This seemingly perfect balance has led some scientists to propose that constants might vary in space and time, with life appearing in regions where the constants have the right values to support it.
String Theory and Extra Dimensions
Newer theories of physics, like string theory, which attempts to unify gravity and quantum mechanics, involve extra spatial dimensions. Changes to these spatial dimensions could be perceived as changes in the fundamental constants.
Dark Matter Interactions
Some physicists theorize that dark matter, which accounts for over 20% of the universe's mass, might interact weakly with atoms' electrons or nuclei. This interaction could cause constants like the fine structure constant to drift or oscillate by extremely tiny amounts.
Searching for Variations in Constants
Scientists have already attempted to measure variations in fundamental constants by studying light from distant quasars. Quasars are extremely bright objects powered by supermassive black holes, and their light can take billions of years to reach Earth.
By analyzing the absorption frequencies of this ancient light and comparing it to current frequencies, researchers hoped to detect changes in constants like the fine structure constant.
However, the results have been inconclusive:
- Some experiments, like those by the John Webb team, observed small variations
- Other research groups found no variation at all
This lack of conclusive evidence highlights the need for more precise measuring devices and potentially new methods altogether.
Enter the Nuclear Clock
The recent breakthrough in detecting the thorium-229 signal is the first step towards building a nuclear clock - a super-accurate measuring device that could be our best bet for measuring variations in fundamental constants.
Atomic Clocks vs. Nuclear Clocks
To understand the potential of nuclear clocks, it's helpful to first understand atomic clocks:
Atomic Clocks
Atomic clocks use the regular oscillations of atoms as their "ticking" mechanism. They work by measuring the frequency of light emitted when electrons in an atom transition between energy levels.
Key features of atomic clocks:
- Extremely stable and accurate
- The second is defined based on cesium-133 atom oscillations
- The most accurate atomic clock (strontium-87) drifts by only 1 second in nearly 40 billion years
Despite their incredible precision, atomic clocks have limitations:
- Sensitive to stray electric and magnetic fields
- Affected (albeit minimally) by temperature changes
Nuclear Clocks
Nuclear clocks would use the energy levels of an atom's nucleus instead of its electrons. This approach offers several advantages:
- The nucleus is shielded from external influences by the electron cloud
- Less sensitive to unwanted electric and magnetic fields
- Potentially 10,000 times more sensitive to changes in fundamental constants than atomic clocks
The Thorium-229 Breakthrough
The main challenge in building a nuclear clock has been finding a suitable nuclear transition that can be excited by available laser technology. Most nuclear transitions require gamma rays, which are beyond the reach of modern lasers.
However, thorium-229 presents a unique opportunity:
- It has a nuclear transition about 10,000 times smaller than typical nuclear transitions
- The transition energy lies in the ultraviolet range, within reach of modern lasers
The Journey to the Nuclear Clock
The path to creating a nuclear clock has been long and challenging:
- 1970s: Discovery of thorium-229's unusual properties
- Early 2000s: Scientists begin considering thorium-229 for nuclear clocks
- 2023: CERN pinpoints the transition's energy at about 8 electron volts
- 2024: Three major experiments make significant progress
- April: German-Austrian team first excites the transition with a laser
- July: UCLA confirms the result, improving precision
- September: Breakthrough using a custom-built ultraviolet frequency comb laser, achieving 1 million times more precision
The Significance of the Recent Breakthrough
The latest experiment has measured the thorium-229 nuclear transition frequency with unprecedented accuracy:
2,020,047,384,005 kHz (± 2 kHz)
This precise measurement is a crucial step towards building the world's first nuclear clock.
Potential Implications of Nuclear Clocks
The development of nuclear clocks could have far-reaching consequences for science and our understanding of the universe:
- Detecting variations in fundamental constants
- Testing theories of physics beyond the Standard Model
- Probing the nature of dark matter
- Improving our understanding of gravity and general relativity
- Enhancing GPS and other navigation systems
- Advancing Earth science and geophysics research
Variations in Fundamental Constants
Nuclear clocks could provide the precision needed to detect even tiny changes in fundamental constants over time or space. This could confirm or refute theories about the nature of these constants and their role in the universe.
Testing New Physics Theories
By measuring fundamental constants with unprecedented accuracy, nuclear clocks could help test theories that go beyond the Standard Model of particle physics. This could include validating or disproving aspects of string theory, supersymmetry, or other proposed extensions to our current understanding of physics.
Probing Dark Matter
If dark matter interacts weakly with normal matter, causing tiny oscillations in fundamental constants, nuclear clocks might be sensitive enough to detect these effects. This could provide crucial insights into the nature of dark matter, one of the biggest mysteries in modern physics.
Gravity and General Relativity
Nuclear clocks could be used to perform more precise tests of Einstein's theory of general relativity. For example, they could measure gravitational time dilation (the slowing of time in strong gravitational fields) with greater accuracy, potentially revealing deviations from Einstein's predictions.
Improving Navigation and GPS
The extreme precision of nuclear clocks could lead to significant improvements in global positioning systems. This could enhance navigation for various applications, from autonomous vehicles to space exploration.
Earth Science and Geophysics
Highly accurate time measurements from nuclear clocks could be used to detect tiny changes in the Earth's gravitational field. This could provide new insights into the planet's internal structure, tectonic movements, and climate-related phenomena like sea-level changes.
Challenges Ahead
Despite the recent breakthrough, there are still significant challenges to overcome before a functional nuclear clock can be built:
- Developing lasers with the necessary stability and precision
- Creating methods to efficiently excite and detect the nuclear transition
- Designing systems to control and manipulate individual thorium atoms
- Addressing potential sources of error and interference
- Scaling up the technology for practical applications
Conclusion
The recent breakthrough in measuring the thorium-229 nuclear transition represents a significant step towards creating the world's first nuclear clock. This achievement is the result of decades of research and collaboration across multiple scientific disciplines.
As scientists continue to refine this technology, we stand on the brink of a new era in precision measurement. Nuclear clocks have the potential to revolutionize our understanding of fundamental physics, probe the mysteries of dark matter, and push the boundaries of what we know about the universe.
While there are still challenges to overcome, the progress made so far is incredibly promising. The coming years are likely to bring further exciting developments in this field, potentially leading to groundbreaking discoveries that could reshape our understanding of the cosmos and the laws that govern it.
As we continue to push the limits of precision measurement, we may find ourselves uncovering new questions and mysteries about the nature of reality itself. The journey towards creating a nuclear clock is not just about building a more accurate timepiece - it's about peering deeper into the fundamental workings of our universe and potentially rewriting the laws of physics as we know them.
Article created from: https://www.youtube.com/watch?v=tXLoBs0ipsI