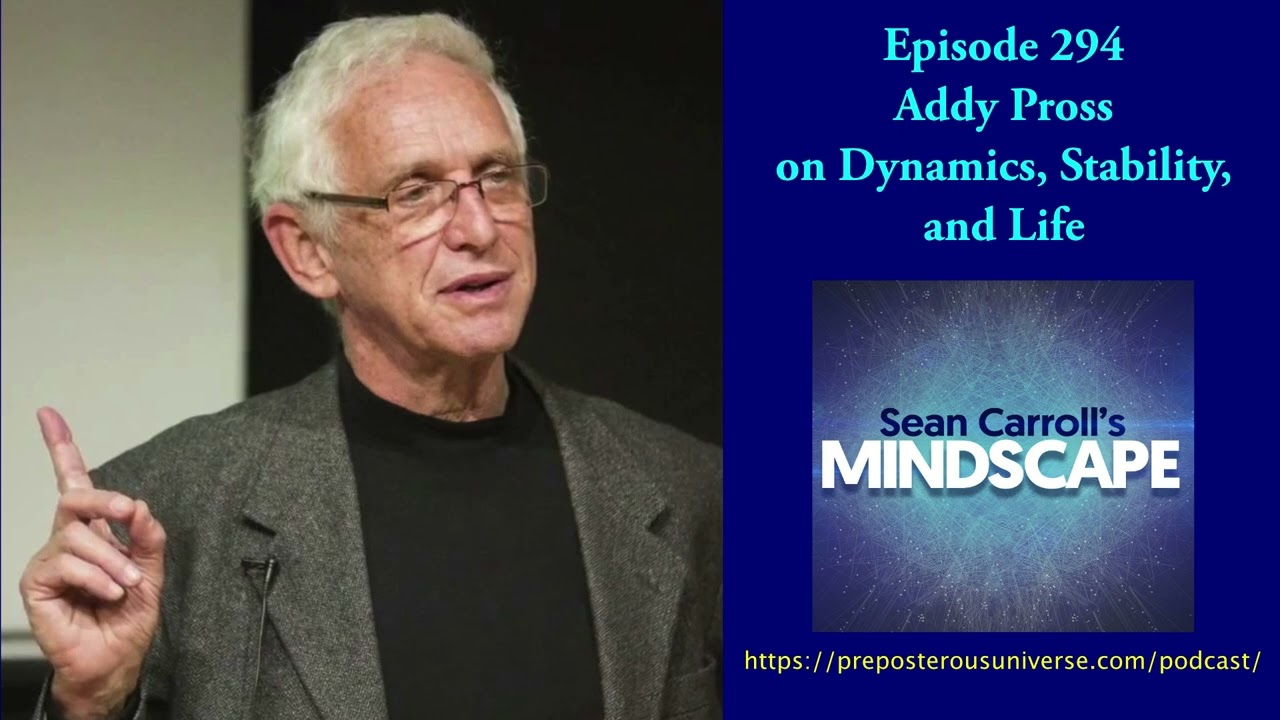
Create articles from any YouTube video or use our API to get YouTube transcriptions
Start for freeIntroduction
The origin of life remains one of the most fascinating and challenging questions in science. While we have made significant strides in understanding the biochemical processes that sustain living organisms, the transition from non-living matter to the first self-replicating entities capable of evolution has remained elusive. Traditional approaches have focused on either metabolism-first or replication-first scenarios, but both face significant hurdles in explaining the emergence of life's complexity.
In recent years, a new perspective has emerged that may help bridge the gap between chemistry and biology - the concept of dynamic kinetic stability (DKS). This approach, pioneered by chemist Addy Pross and colleagues, offers a fresh way of thinking about the stability and persistence of complex chemical systems that may have paved the way for life.
Understanding Dynamic Kinetic Stability
To grasp the significance of dynamic kinetic stability, it's helpful to first consider how we typically think about stability in physics and chemistry:
Thermodynamic Stability
In classical thermodynamics, stable states are those with minimum potential energy. Picture a ball rolling down a hill - it will eventually come to rest at the bottom, in a state of minimum gravitational potential energy. This state is stable because any small perturbation will cause the ball to roll back to the bottom.
Similarly, in chemistry, molecules tend to react and transform until they reach their lowest energy configurations. A crystal of salt or a box of gas at equilibrium are examples of thermodynamically stable systems.
Kinetic Stability
However, there's another type of stability that's crucial in chemistry - kinetic stability. Some chemical species may not be at the lowest possible energy state, but they persist because there's an energy barrier preventing their transformation. Diamond is a classic example - it's not the most thermodynamically stable form of carbon (graphite is), but it persists indefinitely at room temperature due to the large activation energy required for its transformation.
Enter Dynamic Kinetic Stability
Dynamic kinetic stability represents a fundamentally different kind of persistence. Unlike a static, low-energy state or a kinetically trapped high-energy state, DKS systems are constantly turning over, replacing their components, yet maintaining their overall structure and function.
A simple physical analogy is a water fountain. The shape and structure of the fountain persist over time, even though the individual water molecules are constantly being replaced. The fountain is far from thermodynamic equilibrium - the water is being held up against gravity - yet it maintains a stable form as long as energy (in the form of pumped water) is supplied.
In chemistry, DKS systems were discovered relatively recently - only in the past 10-15 years. These are chemical reactions that, when driven far from equilibrium, can spontaneously form complex, self-organizing structures that persist as long as there's a continual input of reactants (which serve as both material and energy sources).
DKS and the Origin of Life
How does this concept of dynamic kinetic stability relate to the origin of life? Pross argues that life itself can be understood as a particularly sophisticated example of a DKS system. Living organisms are far from thermodynamic equilibrium, constantly turning over their molecular components, yet persisting and even replicating their complex structures over time.
The key insight is that DKS systems can potentially provide a bridge between non-living chemistry and the complex, self-replicating systems we recognize as alive. Here's how this might work:
-
Emergence of DKS systems: Under the right conditions (energy sources, chemical feedstocks), simple chemical reactions could spontaneously give rise to DKS systems - complex, self-organizing reaction networks that persist by continually turning over their components.
-
Selection for persistence: Among these DKS systems, those that were better at persisting (by more efficiently using resources or resisting perturbations) would naturally become more prevalent over time.
-
Replication: The crucial step would be the emergence of DKS systems capable of making copies of themselves. This could potentially happen through template-based replication (like RNA) or through the division of self-organizing compartments.
-
Evolution: Once replication is possible, Darwinian evolution can begin. Variations in replicating DKS systems that lead to greater persistence or more efficient replication would be selected for.
-
Increasing complexity: As these systems evolve, they could develop more sophisticated ways of interacting with their environment, processing information, and maintaining their internal organization - gradually acquiring the properties we associate with life.
This scenario potentially resolves some of the challenges faced by other origin of life theories:
- It doesn't require the spontaneous emergence of highly complex molecules like RNA or proteins.
- It provides a natural pathway for the integration of metabolic and replicative functions.
- It offers an explanation for the directionality of evolution towards increasing complexity.
Implications for Our Understanding of Life
The DKS perspective on the origin of life has some profound implications for how we think about biology more broadly:
Rethinking the Role of DNA
The traditional view in molecular biology, encapsulated in the Central Dogma, is that DNA is the master controller of the cell, with information flowing unidirectionally from DNA to RNA to proteins. However, the DKS perspective suggests a more holistic view of the cell as a self-replicating system.
In this view, DNA is certainly a crucial component, serving as a stable repository of information. But it's not the sole driver of cellular behavior. Instead, the cell as a whole controls and modifies its genome in response to environmental conditions. This aligns with recent discoveries in epigenetics and systems biology that highlight the complex, bidirectional flow of information in living systems.
The Nature of Biological Purpose
One of the most striking features of living systems is their apparent purposefulness. Unlike inanimate objects, organisms seem to act with goals and intentions. This has long been a puzzle for biology - how can purposeful behavior emerge from purposeless physical and chemical processes?
The DKS perspective offers a potential answer. By framing life in terms of persistence, it suggests that even the simplest living systems have an inherent "goal" - to continue existing. This provides a foundation for understanding more complex goal-directed behaviors as elaborations on this basic drive for persistence.
The Roots of Cognition and Consciousness
Perhaps most intriguingly, the DKS framework provides a potential bridge between chemistry and cognition. Even the simplest DKS systems must, in a sense, "respond" to their environment to persist. They must take in resources, process them, and maintain their internal organization in the face of perturbations.
This can be seen as a primordial form of cognition - the system is, in effect, "aware" of its environment and its own state, and acts to maintain itself. As DKS systems become more complex through evolution, this basic environmental awareness could potentially develop into more sophisticated forms of cognition and, eventually, consciousness.
This view suggests that consciousness isn't something that suddenly appeared in complex organisms, but rather a more developed form of a fundamental property of life itself - the ability to persist by responding to and manipulating the environment.
Challenges and Future Directions
While the DKS perspective offers intriguing new ways of thinking about the origin and nature of life, it's important to note that much work remains to be done to fully develop and test these ideas:
-
Experimental realization: While chemists have created DKS systems in the lab, we have yet to demonstrate the spontaneous emergence of self-replicating DKS systems from simple starting materials. This is a crucial step in validating the theory.
-
Bridging scales: We need to better understand how molecular-scale DKS processes relate to the behavior of whole cells and organisms.
-
Information processing: More work is needed to elucidate how DKS systems can develop the capacity for storing and processing information in ways that approach biological systems.
-
Boundary definition: Clarifying what exactly constitutes a DKS system and how to quantify degrees of dynamic kinetic stability remains an open challenge.
-
Implications for astrobiology: If the DKS route to life is correct, what does this imply about the likelihood of life elsewhere in the universe?
Conclusion
The concept of dynamic kinetic stability offers a fresh perspective on one of science's most enduring mysteries - the origin of life. By focusing on persistence rather than equilibrium, it provides a potential bridge between non-living chemistry and the complex, self-replicating systems we recognize as alive.
Moreover, this framework has implications that extend far beyond the origin of life, offering new ways of thinking about biological purpose, the nature of the genome, and even the roots of cognition and consciousness. While much work remains to be done to fully develop and test these ideas, the DKS perspective represents an exciting new direction in our quest to understand the fundamental nature of life itself.
As we continue to explore these ideas, we may find that the line between chemistry and biology, between non-living and living matter, is less sharp than we once thought. Instead, life may be seen as a particularly sophisticated example of a more general phenomenon - the persistence of complex, self-organizing systems in a universe governed by the second law of thermodynamics.
This view not only enriches our understanding of life on Earth but also expands our conception of what forms life might take elsewhere in the universe. As we search for life beyond our planet, the insights from DKS theory may help us recognize novel forms of persistent, self-replicating systems that we might otherwise overlook.
Ultimately, the study of dynamic kinetic stability and its role in the origin of life reminds us of the profound interconnectedness of all natural phenomena. From simple chemical reactions to the most complex living organisms, we see the working out of fundamental principles of organization and persistence. As we continue to unravel these principles, we may find that life, in all its diversity and complexity, is a natural and perhaps inevitable consequence of the basic properties of matter and energy in our universe.
Article created from: https://www.youtube.com/watch?v=XIHWldD7Ah0