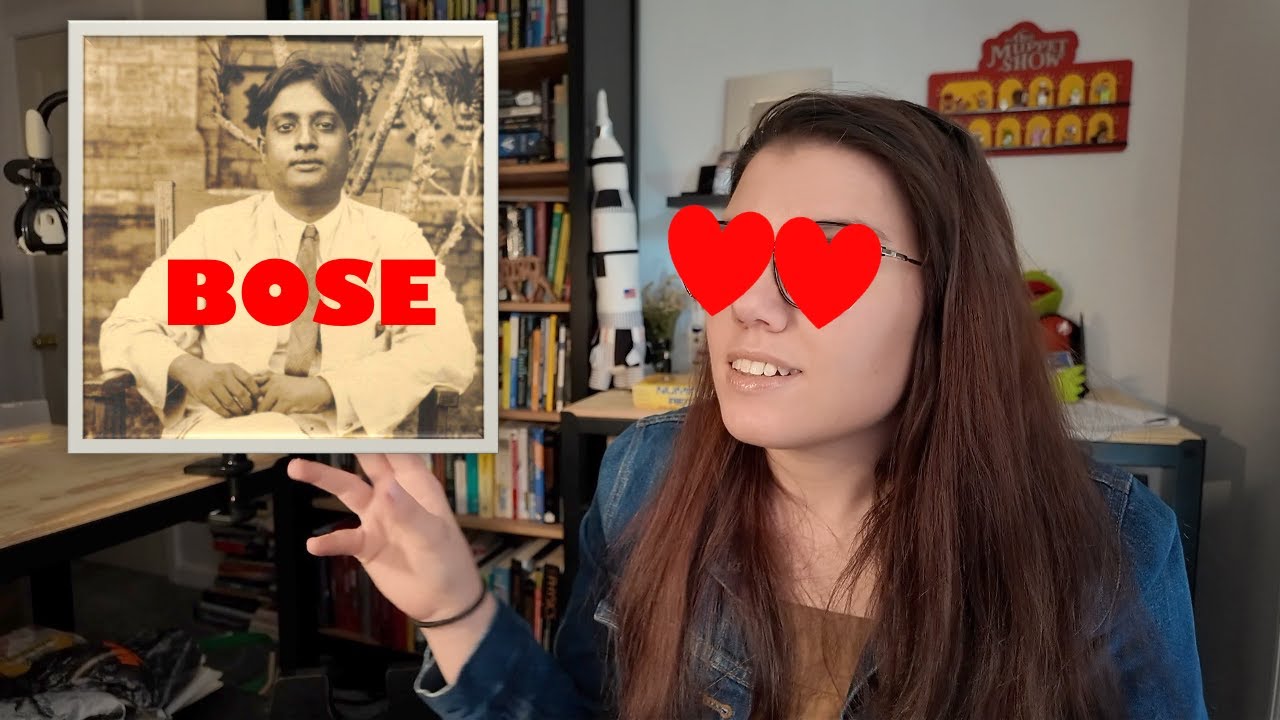
Create articles from any YouTube video or use our API to get YouTube transcriptions
Start for freeThe Origins of Bose-Einstein Statistics
In the early 20th century, physics was undergoing a revolution. The classical theories that had served scientists well for centuries were beginning to show cracks when applied to the microscopic world. It was in this context that Satyendra Nath Bose, an Indian physicist, made a breakthrough that would lead to the prediction of an entirely new state of matter.
Bose was working on a problem that had puzzled even Albert Einstein: how to derive Planck's constant using only quantum mechanics. In 1924, Bose wrote a paper that approached this problem in a novel way. He treated light as a gas of particles (photons) and used statistical methods to describe their behavior.
When Bose's paper was initially rejected by a journal, he took an extraordinary step: he sent it directly to Einstein. Einstein was impressed by Bose's work, recognizing its significance immediately. He translated the paper into German and helped get it published, adding his own notes to emphasize its importance.
The Key Insight: Indistinguishable Particles
The crucial insight in Bose's work was treating photons as indistinguishable particles. This was a departure from classical Maxwell-Boltzmann statistics, which assumed that particles could be distinguished from one another. By making this change, Bose was able to derive Planck's law of black-body radiation purely from quantum principles.
Einstein quickly realized that Bose's approach could be extended beyond photons to other particles. This led to the development of Bose-Einstein statistics, which describe the behavior of particles now known as bosons.
Understanding Bosons and Fermions
To appreciate the significance of Bose-Einstein condensates, it's essential to understand the distinction between two fundamental classes of particles: bosons and fermions.
Fermions: The Building Blocks of Matter
Fermions are particles with half-integer spin (1/2, 3/2, etc.). They include familiar particles like electrons, protons, and neutrons. Fermions obey the Pauli exclusion principle, which states that no two fermions can occupy the same quantum state simultaneously. This principle is crucial for the stability of matter and explains why electrons in atoms occupy different energy levels.
Bosons: The Force Carriers
Bosons, named after Bose, have integer spin (0, 1, 2, etc.). Examples include photons (particles of light) and the Higgs boson. Unlike fermions, bosons can occupy the same quantum state, which leads to some fascinating phenomena.
The Prediction of Bose-Einstein Condensates
In 1925, building on Bose's work, Einstein predicted that at very low temperatures, a large fraction of bosons in a gas could occupy the lowest quantum state, forming a new state of matter: the Bose-Einstein condensate (BEC).
Einstein's prediction was remarkable because it suggested that quantum effects, usually only observable at the microscopic level, could manifest on a macroscopic scale. However, the technology to create such extreme conditions didn't exist at the time, and Einstein's prediction remained untested for decades.
The Long Road to Experimental Realization
Creating a Bose-Einstein condensate proved to be an enormous experimental challenge. The main obstacle was achieving the extremely low temperatures required - just a fraction of a degree above absolute zero.
Advances in Cooling Techniques
Several key developments in atomic physics and laser technology paved the way for the creation of BECs:
-
Laser cooling: Developed in the 1980s, this technique uses precisely tuned lasers to slow down atoms, effectively cooling them.
-
Magnetic trapping: This method uses magnetic fields to confine and isolate ultra-cold atoms.
-
Evaporative cooling: The final step in reaching BEC temperatures, this technique selectively removes the hottest atoms from a trapped gas, allowing the remaining atoms to cool further.
The Breakthrough: First BEC Created
In 1995, after years of effort, two separate teams achieved the first Bose-Einstein condensates:
-
Eric Cornell and Carl Wieman at the University of Colorado Boulder created a BEC using rubidium atoms cooled to 170 nanokelvin.
-
Wolfgang Ketterle at MIT produced a sodium BEC shortly after.
These achievements earned Cornell, Wieman, and Ketterle the 2001 Nobel Prize in Physics.
Properties of Bose-Einstein Condensates
Bose-Einstein condensates exhibit several remarkable properties that set them apart from other states of matter:
Coherence
In a BEC, the atoms behave coherently, acting more like a single, giant quantum object than a collection of individual atoms. This coherence is similar to that seen in laser light, but with matter waves instead of light waves.
Superfluidity
BECs can flow without friction, a property known as superfluidity. This is similar to the behavior of superfluid helium, but occurs at much lower temperatures and with different types of atoms.
Matter Waves
The wave-like nature of particles becomes prominent in a BEC. The condensate can be described by a single wave function, allowing quantum mechanical effects to be observed on a macroscopic scale.
Interference Patterns
When two separate BECs are allowed to expand and overlap, they create interference patterns similar to those seen with light waves. This dramatically illustrates the wave-like nature of matter at the quantum level.
Applications and Research
Bose-Einstein condensates have opened up new avenues for research and potential applications:
Atom Lasers
Just as optical lasers produce coherent beams of light, atom lasers can create coherent beams of matter. These could potentially be used for high-precision measurements and atom interferometry.
Quantum Simulation
BECs can be used to simulate complex quantum systems that are difficult to study directly. This has applications in understanding phenomena like superconductivity and quantum magnetism.
Precision Measurements
The coherent nature of BECs makes them useful for extremely precise measurements. They have been used in atomic clocks and could potentially improve the accuracy of gravitational wave detectors.
Fundamental Physics
BECs provide a platform for testing fundamental concepts in quantum mechanics and exploring the boundary between quantum and classical physics.
Challenges and Future Directions
While Bose-Einstein condensates have proven to be a rich area of study, there are still many challenges and open questions:
Higher Temperature BECs
Current BECs require extremely low temperatures. Research is ongoing to create condensates at higher temperatures, which could make them more practical for applications.
BECs in Space
Experiments with BECs in microgravity environments, such as on the International Space Station, could allow for longer observation times and new types of experiments.
Quantum Computing
Some researchers are exploring the potential of using BECs for quantum computing applications, although this remains a speculative area.
Exotic Quantum Phases
BECs can be used to study exotic quantum phases of matter, such as supersolids, which have properties of both solids and superfluids.
Conclusion
Bose-Einstein condensates represent one of the most striking examples of quantum mechanics manifesting at a macroscopic scale. From their theoretical prediction by Einstein and Bose to their experimental realization seven decades later, BECs have captivated physicists and pushed the boundaries of our understanding of matter.
As research continues, BECs promise to provide insights into fundamental physics, potentially leading to new technologies and applications. They serve as a testament to the power of theoretical physics to predict new phenomena and the ingenuity of experimentalists in bringing these predictions to reality.
The story of Bose-Einstein condensates is far from over. As we continue to explore this unique state of matter, we may yet uncover new surprises and applications that could reshape our understanding of the quantum world and its connection to the macroscopic realm we inhabit.
Article created from: https://www.youtube.com/watch?v=GX-fFXbT0rY